Greetings, everyone, welcome to the new blog post focused on a great topic – The mechanical properties of metals. It is necessary to know about the mechanical properties of metals for engineers, designers, and everyone who has an interest in material science. In this post, readers will get to understand the relationship between the mechanical properties of metals and the suitability of metals in different fields.
We’ll also talk about factors that govern the mechanical properties of metals, and you stand to benefit in that you will be in a better position to select the right material to be used for your particular job. Welcome to a post in which we will demystify the mechanical properties of metals and establish their relevance in the modern world!
Table of Contents
Introduction to Mechanical Properties of Metals
The properties of metals are important, especially for mechanical engineers and metallurgists in their areas of practice including aerospace engineering, automobile engineering, civil engineering, and production engineering. This knowledge aids in the selection of suitable materials for application and also in winning confidence that the material in use will function appropriately under load. Some of the basic mechanical properties are ultimate strength, proof strength, hardness, elongation, and impact strength. In this post of “Mechanical Properties of Metals,” we will discuss each property in detail including factors affecting them, the methods used in testing them, the various applications, and potential trends in further study of metals.
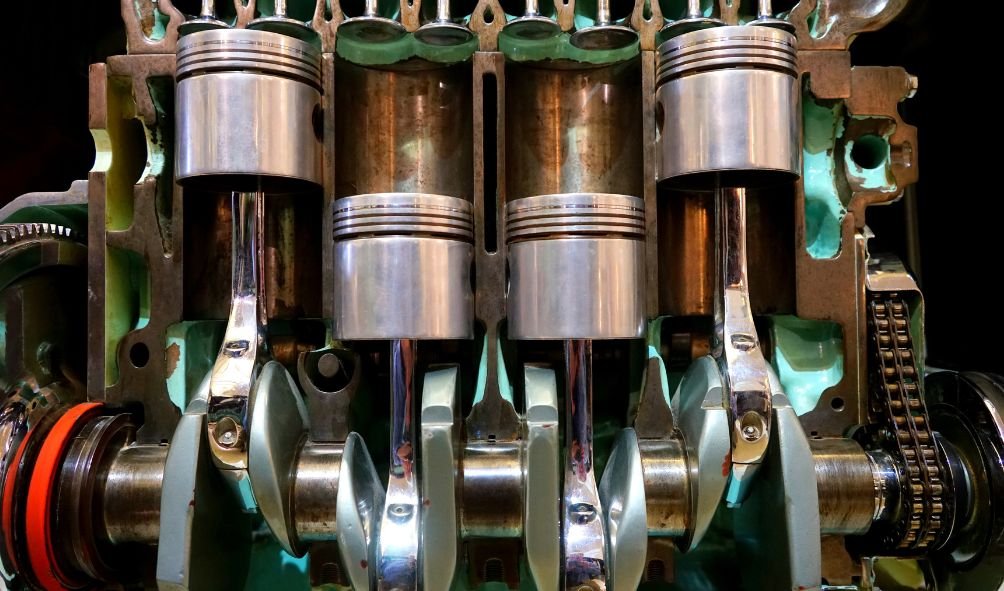
Fundamental Mechanical Properties
2.1 Tensile Strength
Tensile strength is defined as the maximum stress within a material that it can withstand while undertaking direct tensile test stress. This property can be determined by pulling an original sample to failure while applying tensile loads to indicate the strength of the material. It is measured as stress i.e. force/area, in Megapascals or pound per square inch (psi).
There are two key metrics associated with tensile strength:
- Ultimate Tensile Strength (UTS): This is the measure of stress it’s possible to apply to a material before it reaches its maximum elongation. After this point, the material may decrease in cross-sectional area and ultimately fail.
- Yield Strength: This is the stress at which a material changes its shape through a permanent deformation. In most of the metals, this point is significant because the value within this region symbolizes the onset of deformation.
Tensile strength is important in any case where there is applied some sort of stretching forces and here, we can predict suspension bridge cables and building structures, for instance.
2.2 Yield Strength
Yield strength is an essential material parameter that defines the boundary of the elastic deformation of the material. It is the stage at which material deforming under load is no longer elastic when the load is removed but has to undergo plastic deformation.
The yield point can be influenced by several factors, including:
- Material Composition: Various alloys possess dissimilar yield strength characteristics. For example, its Minerals Specialty Products division offers bronzes that have better yield strength for their desired applications for a given collection.
- Temperature: It was ascertained that the yield strength of a material reduces as the temperature increases. As the temperature increases, metals are more likely to become ductile in the sense they deform easily.
- Strain Rate: It is also evident that the rate of deformation can influence the yield strength of a material. Higher strain rates also lead to higher yield strength because more resistance to deformation has been offered.
For engineers to be able to design structures that would effectively withstand the vice, it is important for students to understand yield strength.
2.3 Hardness
The strength of hardness is a measure of a material’s resistance to localized plastic deformation, dents, or scratches. It is a very valuable property that oftentimes correlates with the resistance to wear. Sometimes, when wear resistance is critical-for instance, cutting tools, gears, and bearings-a certain degree of hardness can be important as well.
There are several techniques to measure hardness:
- Brinell Hardness Test: This is the impression created by making an indentation on the surface of the test piece with a hard sphere and measuring the diameter of the impression.
- Rockwell Hardness Test: The depth of the indenter penetration under a large load as compared to the penetration made by a preload. It is directly readable through a dial.
- Vickers Hardness Test: Similar to the Brinell test. A diamond pyramid indentation is used; thus, it gives a continuous scale of hardness numbers.
Hardness depends significantly upon several factors including:
- Alloying: Elements such as carbon have a tendency to increase the hardness and introduce hard phases like martensite in steel when alloyed with iron.
- Heat Treatment: Quenching, tempering, etc., changes the values of hardness to give material properties for a specific application.
2.4 Ductility
Ductility therefore is defined as the ability of a material to undergo a large amount of deformation and still not fail. It is a valuable property in operations that involve bending or manufacturing of the material in various industries including metal forming.
Ductility is often quantified by two primary measures:
- Elongation: This is determined by the proportionate or percentage increase in the elongation of a test piece before its fracture during a tensile test.
- Reduction of Area: Minor to moderately severe cross-sectional area reduction reflects the extent of the fracture.
Factors influencing ductility include:
- Temperature: In most cases, it has been observed that ductility is improved when heated, meaning that metals in the forms can be easily formed.
- Grain Structure: The refinement of structures leads to higher ductility due to higher grain boundaries through which displacement occurs.
Ductility is important in making certain that components can effectively handle energy and set components into shape and not shatter easily in dynamic applications like automobiles.
2.5 Toughness
Toughness is the capacity of a material to resist deformation plastically without rupturing when absorbing energy. It is a vital property that complements both strength and ductility, particularly in terms of applications that undergo impact or shock loading.
Toughness is often tested by impact tests, such as the Charpy and Izod tests. These tests describe the energy that must be absorbed by the material during fracture. The factors contributing to toughness are primarily:
- Temperature: Increasing the temperature tends to increase toughness. Lower temperatures tend to embrittle materials, leading to fracture.
- Microstructure: Some microstructural characteristics, for example, fine grain size or specific phases can enhance toughness.
- Loading Rate: Material can sometimes have different toughness values with regard to loading rate. Higher loading rates can sometimes decrease toughness.
Toughness is quite essential in applications that involve bridges, pipelines, and structural components where sudden impacts or changes in load may be experienced.
Influential Factors in Mechanical Properties
3.1 Temperature Effects
Temperature has a dramatic influence on altering the mechanical properties of metals. As the temperatures continue rising, the atomic vibrations will increase and sometimes induce:
- Increased Ductility: Most metals have improved ductility when heated high; this means these metals can be more easily shaped and molded.
- Decreased Yield and Ultimate Strength: The increase in temperature will in general decrease yield and tensile strength; hence, many materials are used inadequately in such hot environments.
At low temperatures, metals can become brittle and may fail catastrophically. The temperature dependence of mechanical properties is therefore important for any material used in extreme environments, including those encountered in cryogenic applications or high-temperature applications.
3.2 Alloying Elements
Alloying elements are added to a metal to enhance properties. A few of them are listed:
- Carbon in Steel: Carbon addition increases hardness and strength; such steel is used for structural applications.
- Nickel and Chromium in Stainless Steel: Ni and Cr have resulted in increased resistance to corrosion and toughness when used in chemical processing.
Alloying elements can have substantial influences on properties beyond strength and hardness. Ductility, toughness, and fatigue resistance have some impact as well. In fact, the uniqueness of each alloying element impacts the overall performance of a given metal to means that it is possible to design specific properties according to need.
3.3 Microstructure
Metals possess grain size, phase distribution, and defects as significant factors in control of their mechanical properties. Some of them are discussed below:
- Grain Size: Lower strength and toughness for small grain size (Hall-Petch relationship) and, therefore, finer grains generally tend to improve strength, ductility, and toughness.
- Phase Composition: Many phases in metallic matrices like ferrite and cementite in the case of steel produce properties that would be compared with those of various individual phases. For instance, the introduction of hard phases into relatively softer matrices often improves toughness significantly.
- Dislocations: The motion of dislocations (line defects in the crystal lattice) is responsible for plastic deformation. The density and distribution of dislocations can significantly influence yield strength and ductility.
Knowing how microstructure is controlled by heat treatment, alloying, and mechanical working makes it possible for engineers to design materials with properties optimized for a particular application.
Testing Methods for Mechanical Properties
4.1 Tensile Testing
Tension testing is the most widely used technique for the determination of the mechanical properties of metals. A standard geometry test specimen is pulled in tension until a fracture occurs, and the data obtained give information on tensile strength, yield strength, elongation, and modulus of elasticity.
In tensile testing, results are generally found to fall into a form of stress versus strain curve that shows stress, force per area against strain, and deformation. Main points on the curve include:
- Elastic Region: The initial linearly proportional part of the straight-line section describing the relationship between stress and strain.
- Yield Point: This is termed as onset where deformation begins to be permanent in nature.
- Ultimate Tensile Strength: This describes the highest point on the curve which depicts the maximum stress value before the rupture.
- Fracture Point: It is actually at the point when the material breaks.
Tensile testing: This is essential information for the choice and design of materials which hence helps understand the material behavior under various loading conditions.
4.2 Hardness Testing
Hardness is the resistance of a material against localized deformation. The selection of the hardness test depends upon the material and application:
- Brinell Hardness Test: Applied to a softer material, where a hard steel or carbide ball is pressed onto the material under a specific load.
- Rockwell Hardness Test: It is widely applied in industry due to the fast results obtained with minimum pre-treatment of the sample. Several scales (A, B, C) are available for different types of materials.
- Vickers Hardness Test: Applicable to both hard and soft materials, since they measure the size of an indentation formed by a pyramid of diamond.
These tests, as with those already described, are fine sources of very useful data for estimating wear resistance and correlate well with tensile strength and ductility making these a valuable contribution to quality control.
4.3 Impact Testing
Impact testing measures the toughness by quantifying the response of the material when sudden loads are applied to it. The most commonly used methods are the Charpy and the Izod tests, measuring the energy absorbed at the fracture point:
- Charpy Test: A notched sample is hit with a pendulum, measuring the energy at the instant the sample breaks. The Charpy test is generally used when evaluating toughness in low-temperature materials.
- Izod Test: This is another impact test similar to Charpy except with the vertical notched specimen. It can always be carried out on materials whose geometries are more complicated than other samples.
Impact test results are used to predict the material behavior under practical conditions when shock loading or impact loads may occur.
Applications of Mechanical Properties in Engineering
Knowledge of the mechanical properties of metals is essential during the selection of materials for various applications of engineering:
- Construction: The tensile and yield strengths are relevant to determine if the materials are suitable for structural parts, such as beams and columns, in bridges and buildings.
- Automotive Industry: Ductility and toughness have the utmost importance in crash components since they absorb the energy of impact to reduce passenger harm during collision. High-strength steels are often used for their favorable properties.
- Aerospace: Materials should be lightweight as well as strong because the structures of aircraft are made from them. For the aerospace industry to run, it requires those alloys that possess superior strength-to-weight ratios plus resistance to fatigue and corrosion.
- Manufacturing: Forging, casting, and machining are the processes where materials have to be of adequate hardness and ductility so that they do not collapse during forming.
Mechanical property knowledge is one of the ways with which the engineer will design safe, efficient, and cost-effective systems to meet the demands of their applications.
Conclusion and Future Trends in Metal Properties Research
Metal properties research is constantly changing as demand for better-performance materials increases, and the pace of technological development is tremendous. Future trends are:
- Nanotechnology: The combination of nanoscale and structured materials has led to better mechanical properties such as strength and toughness coupled with lower weight and significantly long ranges of applications.
- 3D Printing: Additive manufacturing 3D printing is changing the metal production landscape with its option to offer complex geometries as well as to provide tailored mechanical properties by controlling microstructures precisely.
- Materials in Motion: Research and the development of materials that would respond to environmental changes, like temperature or stress, do progress and may, at some point, find new applications in aerospace, biomedical, and structural fields.
- Sustainability: Alloy and process development to replace recycling will be the key orientation of the future and lead to more environmentally friendly research and development, still with or even better performance.
In summary, control of mechanical properties of metals will remain at the heart of materials science and engineering, and new achievements in these fields will stay devoted to and address social challenges.
Thank you very much for visiting our blog post “Mechanical Properties of Metals”
Thank you for reading our blog post on the mechanical properties of metals. Hope you found this information on the mechanical properties of metals very informative, and worth using for your projects and interests. The mechanical properties of metals have to be understood well to make informed decisions about engineering and design.
It should be noted that the performance and suitability of metals for any particular application largely depend on the mechanical properties of these metals.
Upon probing the mechanical properties of metals, you should be able to understand every influence exerted on those properties whether it is strength, ductility, or anything in between. The end result for people is better designs and innovations in almost every domain.
Thanks to readers, and thanks for your interest in the mechanical properties of metals; we look forward to exciting topics here with you shortly. Once again, we thank you for participating in our exploration regarding the mechanical properties of metals, and we look forward to seeing you here again soon as we continue to dig deeper into the fabulous aspects of the mechanical properties of metals!